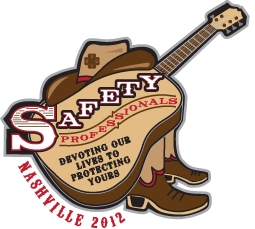
SOLAR BOILERS: EVOLVING ISSUES WITH AN EVOLVING TECHNOLOGY
The following presentation was delivered at the 81st General Meeting on May 14, 2012, by Don Cook and Tim Zoltowski. It has been edited for content and phrasing. The accompanying slide presentation can be viewed here.
Speaker Bios:
Solar boilers are becoming more and more plentiful, especially in the United States. While these boilers are generally seen in a positive light, there is more to this technology. Don Cook and Tim Zoltowski have studied solar boilers and have come up with interesting perspectives.
Don Cook is principal safety engineer for the Pressure Vessel Unit of California's Department of Industrial Relations. Overseeing one of the largest National Board jurisdictions in North America, he possesses more than 25 years of experience in the pressure equipment industry. Don earned his degree in mechanical engineering from Cal Poly. Starting his career in 1984 as an engineer for a private company, Don later joined the state in 1989 as an associate engineer. He was named principal engineer in 2003, and was elected to National Board membership that same year. An active member of the California Boiler Inspectors Association, Don possesses A, B, and N endorsements.
Tim Zoltowski is regional risk engineering manager for Zurich North America Insurance. Mr. Zoltowski has managed machinery breakdown engineering services for Zurich for the past seven years. More recently he has been involved in loss prevention consultation efforts for the use of concentrated solar power to generate steam.
Mr. Cook:
I would like to begin my presentation with a description of the phone call that all of us chiefs dread; a phone call our office received in October 2008. I didn’t know the company, but a fellow said, “Umm, we’ve got this thing we built down in the Bakersfield area and we are about finished with it. Didn't build it to ASME code, and we’ve got Governor Arnold Schwarzenegger coming out next week to take a look at it.”
And so I'm thinking, what have they built down there? Sure enough, it was what we suddenly called a solar boiler. And so, as the jurisdiction, how do you handle a new piece of equipment that comes into your state? And what the heck is a solar boiler?
Is it a fired boiler, like we are accustomed to seeing, with flame and natural gas or coal, impinging on some tubes? Or is it an unfired pressure vessel, more like what we would see in a refinery where processed gas goes through tubes? Is it a forced flow steam generator? Is it a series of pipe with water flowing inside of it? Is it Section 1? Is it Section 8? Is it B-31.1?
What is the particular law in your state? Does your state define a boiler as being something that is fired, or is it a boiler because of the application of heat? And how do you build something like this to code? Because no one had ever built a solar boiler in California before, at least they hadn't in 30 years.
So this is the phone call I get, and I'm scratching my head: what in the world am I going to do with this thing? Obviously we received a whole slew of drawings and calculations from the company. I started talking to my boss at the division level, who talked to his boss at the department level, who was going to talk to his boss at the agency level, who talks to the governor.
So we had a whole series of events taking place. It was going to fall on our shoulders to make the determination within a week's time as to whether or not this was going to be suitable for use in our state.
Surprisingly, there are many different types of solar boilers out there. The standard type we’ve seen over the last 30 years might have been mounted on top of your roof at the house to make hot water for your home. That would be a standard parabolic trough. It focuses the sunlight right on the tube that runs down the middle of it, goes down to your hot water heater, and cycles through – a standard design.
The newer type is a Fresnel mirror, which is essentially a series of flat mirrors that project sunlight back up on a set of tubes that run overhead maybe 50 feet in the air. The more common design you might see and what Tim is going to talk about in more detail, is a mirror field that might be a square mile of flat mirrors that move with the sunlight during the day, focusing the sun's rays up onto what would look like a standard boiler except for the fact that it's standing 200-250 feet in the air. And surprisingly, just from the application of sunlight, you can get both saturated steam all the way up to superheated steam from a solar boiler.
Sunlight can produce a significant amount of energy. These plants can be a complete power plant design creating megawatts of electricity, or we have seen designs for rooftops that would help run the HVAC system for a building, creating cooling or heating as needed for your particular office environment.
These are types of standard designs, but many people have come into my office over the last three years with what I generally call a whacky idea because I have never seen them before trying to create steam. And it's a steam boiler. How do we incorporate that concept into our jurisdictional rules and regulations?
There are different ways of transferring heat from sunlight. One way is having regular water flowing through the tubes, and as the sun reflects on it, the water gets hotter and hotter as it goes through the system until it eventually becomes steam. And if you let it go even longer and control the flow just right, steam can become superheated. That certainly is a potential, because what you are looking for on the outlet side is to be able to run a turbine. You generally want superheated steam.
Another methodology is to use some kind of thermal fluid heating oil that goes through the tubes out through the solar field, travels back and forth until it gets hot enough, and then it runs through like a standard heat exchanger. It goes through a heat exchanger, creates steam on the other side, and then you use that steam to create power.
A third type we see nowadays is molten salt. Molten salt flows through the field and slowly travels through the system until it gets hotter and hotter and hotter. It will run through a heat exchanger and produce steam. The unique aspect of molten salt is that it will retain heat throughout the evening.
Energy consumption typically peaks around 5:00 p.m. Businesses are still in operation, people come home from work and turn on the air-conditioning or start running their driers, etc. So our energy consumption peaks right around 5:00 in the afternoon, but our solar power has started to fall off by then. Sunlight goes down and you don't have as much energy. The theory is that molten salt will retain heat for a good portion of the evening, creating more power throughout the day.
And you can use the steam either externally -- we have one in the oil fields in the Bakersfield area that dumps steam into the ground to help produce the oil, like we have currently with regular steam gas-fired plants. And then the other is to drive a regular standard turbine producing electricity. Those are the common ways we would utilize the steam from solar boilers.
So this brand new design came into my office, and I wondered how we were going to make sure it’s safe. What kind of safety concerns could we have? The designs are essentially new and somewhat experimental. We didn't know what to expect. I didn’t have a code book to go to with specifics for these types of designs.
We treated the solar flux the same as a flame. If it’s the application of heat, you get the same thing, so we looked at it just like we would on a standard boiler and called the solar flux a flame.
But then we wondered, what is the low water cut-out on this thing? The industry says we can turn the mirrors so they face downwards. So part of our system was to determine if they could defocus the mirrors quickly enough to where there was no overheating on the tubes; and see if the controls would defocus the mirrors in a short period of time.
One of the designs was such that if the mirror was locked in place (didn't move at all) as the sun moved through the sky, it would take about six minutes for it to be defocused, and the theory was that there was not enough residual heat in the time frame to melt the tubes. If we had a low water condition and the power went off and the mirrors would not move, in six minutes they would defocus and be shooting out in the sky and our tubes wouldn't overheat.
But how hot can the solar flux be? I don’t get sunburnt when I go outside. But if you reflect the sun off of a mirror, it can get over 2,000°F and melt the steel support structures if you are not careful in the design of solar plants.
Then we started thinking about how to handle the inspection requirements. These are in cyclical service: they go up in the morning, they come down in the evening, and 365 days a year we cycle these large-scale boilers on and off. We do that with dry cleaner boilers, but they throw those things away after a little while. What kind of long-term damage could we have? What kind of concerns do we have for these boilers 20 years down the road?
In my position I always consider the guy that comes after me. Because I'm looking at things somebody else decided upon 30 years ago. I know somebody is coming after me in 30 years. How are we going to handle these kinds of jurisdictional issues? Is this a fired boiler? Our boiler rule in California is such that it's the application of heat that defines a boiler. So that told me it was a boiler. And you feel sorry for the manufacturers. They are making something new. How do they bring it in within the confines of our state laws and ASME code? How do you address those issues?
We came up with a code case and utilized ASME Code Case 1855-1. It was my understanding it was originally used for HRSGs when you have a Section 8 component within a Section 1 boiler. And our desire was to make sure that somebody took responsibility for the entire apparatus, all the feed water pumps and the economizers, all those things we would look at in a standard boiler. Using that code case allows you to bring in all the various pieces and call that part of Section 1.
We also could have used a refinery-type idea where you have a heat exchanger that uses processed gas to come through and create steam for use within the facility. But no one ever takes complete responsibility for a boiler design in that scenario. So we felt the use of the Code Case 1855 was certainly worthwhile and applicable in this situation. And the irony: on a Friday morning when I showed up at the office, I pulled up my e-mail and there was a message from somebody in Spain who said they were going to build a solar boiler in the Mojave Desert, and they wanted to use Code Case 1855 to do it. I said, “Holy-moly, I got one right for a change.” Somebody else was thinking along the same line that we were. It was encouraging.
ASME's solution? They created a subgroup on solar under Section 1. It allows all the experts within the industry to make those decisions on what needs to be addressed. When someone calls me interested in solar, I refer them to ASME Section 1, as it will point them in the right direction.
Conclusion: Jurisdictional people like us love a design code such as ASME's so we can get consistent rules throughout the industry and not have to come up with them by ourselves. That's where Code Case 1855 came in handy for me. Most jurisdictions have the ability to accept something as equivalent to ASME code, either through the chief, which I have that authority in California, or through a boiler board.
We decided to treat a solar boiler like any other boiler: requires annual inspections, requires permits to operate, and requires ASME code construction. And similar to the nuclear and hydrogen industry, the solar industry cannot afford to have an accident. We hope we provide guidelines to the manufacturers and others who are involved in this industry so that everyone knows it's a level playing field and will have the opportunity to build their solar boilers accordingly.
MR. ZOLTOWSKI
I’d like to widen the scope and discuss some factors that may affect the useful life of the equipment, and talk about business continuity. It might seem odd to some of you that we started this presentation on solar boilers by talking about emerging technology. If you are familiar with the legend of Archimedes, then you might understand that the application of concentrated solar power may have been put to practical use as early as the Second Century A.D. when it is said that Archimedes used reflective glass to focus the sun's energy on the hulls of wooden ships during the siege of Syracuse, setting them ablaze. Whether or not the legend is true, we know that over the years there have been many scientists that have tried to prove or disprove the plausibility of that legend. And it's the interest in this technology that has bled over to trying to figure out a way to use it for commercial application.
One of the biggest pushes for developing this technology is times when gas or oil prices increase. The initial push for developing this technology happened in the mid-1970s. Parabolic troughs, the parabolic dish, and linear reflectors began to be developed. Over the years it turned into proven technology. We have seen this again as recent as 2010 when we really started to see the research focus on power tower technology.
As we focus on power tower, we will see that the push to develop this technology comes from the promise of a couple different things: higher output capacities to get superheated steam for power generation, higher peak operating temperatures, and greater efficiency. The steam we produce in the power tower can be used for any application we want. Let's assume we are going to use it to generate power. And there is a good reason to do that. If we use concentrating solar power to generate electricity, it may qualify us for some subsidies for renewable energy, depending on where we are and what government has authority.
A typical arrangement of a power tower includes three main components. We will discuss two of these three components. The first is the field of heliostats, and this is what concentrates power on a point in space. The second is the receiver, which receives the concentrated energy. And the third is turbine. We won't spend a whole lot of time talking about turbine. There is nothing different in it than conventional steam turbine technology. I only mention it to set the context of our discussion.
The heliostat field is an arrangement that can be any geometric shape you can think of. A field of mirrors is controlled by servos operated by a computer program. The computer program is updated nightly so that the mirrors will track the sun on its ecliptic the next day. Each mirror is controlled individually by its own servo motor and they move to focus, concentrate, or multiply the sun's energy on the void in space, and of course, we hope that that point in space is the receiver. At least, that's the design of it.
The receiver is nothing more than an adaptation of a typical water tube boiler, and we have discussed that already. One big difference is that the water tube boiler is supported 165 feet in the air on the top of a tower, and it weighs about 65 tons. I give you those two parameters because both of them can be interesting at different stages of developing this technology. In the construction phase, thinking about the fact that this adaptation of a water tube boiler weighs 65 tons can give us some concern when we think about having to lift it with a crane and set it on top of a tower 165 feet in the air. And 165 feet happens to be under the height that would cause us trouble, but the higher you place the receiver, the smaller your heliostat field can be. So there is a push to lift those higher and higher, and in many places, if you get over 200 feet, extra permits must be put in place because of air traffic. Both of these issues need to be taken into consideration in the start-up phase of one of these plans.
The water side is the same as you would expect from any normal water tube boiler. It has a normal water level and a steam water line, and the differences only occur on the side that we would normally call the fire side. Before we get into the issues that may exist here, I want to talk a little bit about the return on investment. I think it's interesting to note that the operating costs for a plant that uses power tower technology are pretty close to the same as conventional steam plants. But there are two big differences. Number one, the start-up costs today are three times what it typically takes to start up the same capacity steam plant, but there are no fuel costs. So that's how we would calculate a return on investment, to balance those two together and figure how long it would take to pay that three times the cost. I understand each time a new plant is erected, it happens quicker and cheaper. So I think that three times the cost will eventually come down and this will be a much more viable method.
To understand the issues that affect the useful life of the equipment, let's first talk about the operating environment. The idea behind a solar boiler obviously is to maximize the amount of solar thermal energy that can be concentrated on the receiver. To do that, most likely they are going to be located in a desert climate. This maximizes the thermal reflection because those climates will minimize the things that tend to attenuate thermal energy, such as cloud cover and pollution. But even though this is the optimal operating environment, there are challenges that come with it that may pose some potential hazards to widespread commercial use.
The wind in the desert, especially in the evening, can be quite severe. When you combine wind with the dusty conditions, it can be very harsh on the heliostat field. It can cause damage to the mirrors, or at the very least, it can dull the surfaces of the mirrors and reduce the reflectivity which will in turn reduce the efficiency. Also in desert climates, the hours of darkness tend to be much colder than daytime, and it's almost impossible to maintain the energy that's developed during the day and there is a rapid loss of stored energy.
On a typical distribution curve for solar thermal energy, the amount of thermal energy and the time of day will vary depending on the time of year and the latitude and longitude. There will be a point in time as the sun comes up from behind the horizon that the thermal energy will begin to rise. Then there will be a point in time somewhere near meridian height that will peak in the energy. Then there will be a point in time when the energy levels will reduce to a point where we can no longer produce steam as the sun disappears again behind the horizon. At some point in time as the energy increases, we will begin to produce steam, and then at some point in time we will no longer produce steam. So let's keep this curve in mind as we talk about these issues, because it's those two points, the beginning and the ending, where we are going to find our first issue.
If we think about this as a power generation facility, we can see that production demands will put pressure on reaching that point in time when we can produce steam as quickly as possible, because it's not until we produce steam that we can start earning money. This production demand could cause us to focus as many of the heliostats on the receiver as possible so that we can reflect as much energy as possible. We might do that without regard to the heat-up rate, which can be dangerous.
If we look at heat up verses cool down, we know that heat up is the more limiting of the two temperature transients. If we put enough pressure on productivity so that we don't care about heat-up rate, we can overstress the metal. Cool down is another thing to be concerned about. Cool-down rate isn't as limiting from a stress standpoint as heat up is. But when we think about why it's happening, we can't control it. At least on the heat up, we can control that rate by either focusing or defocusing the mirrors. But when the sun goes down in the desert, the desert decides how quickly it's going to take the heat away. We can't control the speed at which we lose energy.
When we shut the boilers down at night, like Don said it happens every day and every night, we secure the flow, which will help maintain some of the stored energy. But if you think again about the receiver being 200 feet in the air (we cool it rapidly in the desert air) that sets up natural circulation. It works against us to get rid of all that stored energy.
Many who have used these types of boilers have experienced heavy scaling and some oxygen corrosion. When you trace it back, the root cause is because if we use a typical chemistry control program on this boiler water, it's not going to address the concerns we see because of the frequency and the rapidity of heat up and cool down. And at night when we get very low temperatures, it renders our DA tank ineffective. In the morning we have a much higher level of oxygen in the feedwater just with the normal make-up feed rate than we would desire, and so we have to design a chemistry program that not only takes care of the cyclic nature of the heat up and cool down, but also the increased oxygen that's in the feedwater.
One solution can be to use a secondary gas-fired boiler that will maintain an operating temperature in the DA tank overnight and keep the oxygen levels down. But if we take that route, we need to make sure it's not affecting the renewable energy status I talked about earlier, because it could remove our qualification for those grants if they existed. Basically, we are talking about a water tube boiler again. As Don mentioned, it remains important for us to conduct the internal inspections. And that becomes particularly important when we think about the susceptibility these boilers have to corrosion and scale.
But the design of solar boilers doesn’t make that easy. The construction does have manways, so we can crawl into the water side of the boiler. But again, it's 200 feet in the air. In order to get in there, it would require hanging over the edge of the platform in a harness, and I'm not sure how many of us would be real eager to do that. In lieu of a physical internal, many times a borescopic examination is used, which I think is a viable alternative as long as we make sure the operator of the borescope is qualified, and also the inspector of the boiler can read the results as well.
These issues need to be taken into account when designing an inspection program for solar boilers. And wind can be our enemy in both of those circumstances, because when wind gets to a certain level, it precludes the entry into the tower from a safety standpoint. If we are in a shutdown and we have an internal plan and the winds are too high, we won't be able to do either one of those, a physical internal or a borescopic examination; therefore, careful planning needs to go into the outages to make sure we can get the internal completed.
And lastly, from a safety standpoint, lockout and tagout need to be considered. Although Don has made remote control stations illegal in California, in most applications of power tower facilities, the control of heliostats is remote. When the one in California was built, workers were in the tower, and at random one of the heliostats focused on the tower, and it was noticeably uncomfortable with just that one focused on them. So it wouldn't take that many before it became a very dangerous situation.
Overheating is a concern that can result from transients that come from either wind or cloud. We have talked about the control of the servos of the heliostats that track the sun as it moves across the sky, and that control program assumes there is very little attenuation of the energy. When we have cloud transient or overcast skies, this is the largest attenuation we can see of that energy. And the way it's compensated for is by monitoring the boiler parameters, and when a cloud cover is detected, it will increase the number of heliostats that are in focus in order to make up for the energy loss and maintain a high enough energy level to produce steam. That seems like a pretty good solution until you think about a transient. Because if we are not maintaining a relatively steady attenuation factor, then what happens is as the cloud cover forms, more heliostats come into focus.
When the cloud cover clears, we now overheat the boiler in a very short period of time. In addition to the programming, and detection of overcast skies, there needs to be a detection program that will detect transient. In a research and development situation, the safest thing to do is shut the boiler down by placing the heliostats in their safe position and then restarting it according to the normal procedures. But if this is a commercial application that's generating power, that may not be a viable solution. It needs to be programmed effectively so that it won't overheat the boiler but maintain the highest level of energy focused on the receiver at all times. It's critical to have a trip position programmed in, like Don mentioned, so if there are situations that we can't control or don't understand, we can trip the mirrors into a position where none of them are focused on the receiver, and that's the safest possible solution.
One main issue that still stands in the way of widespread commercial use is the limited time of useful steam production. If we think about all the issues that we talked about so far, we can ensure that we generate steam as early in the day as possible, and we can protect ourselves from damage from the high heat-up rate in the process. We can make sure the heliostats are clean to maximize reflectivity. In fact, there are robots designed to do that on a daily basis. But one thing we can't change is the number of hours the sun is shining during the day. There is research into methods to capture and store heat and minimize at least the heat loss that happens over the dark times, and one of the most promising is molten salt.
If we go back to that original distribution curve, we can see those hours of peak electrical use are the hours we're ramping up and we're shutting down, and that's precisely the time we are starting to generate enough steam to produce power when we need the most electricity. So if we applied a technology like molten salt, what it will do is push that curve out on both sides and allow us to generate steam earlier in the day and for a longer period of time to take advantage of those peak times of energy use.
In conclusion, if we think about the commercial application of concentrated solar power in especially power tower technology, this is something that's going to continue, and I think it's something that's going to continue at a much higher and increasing rate. We need to address this situation about standardizing the designs, maintenance, and operation of these units. If we do that today, that's our choice. If we wait until accidents occur, we will be doing it forced by that accident.
Much has been accomplished since 2010 in developing this technology, but I think we are still out in front of it from a safety concern. And so my urge today is that we stay out in front and not respond to statistics.
Q&A
MR. BERGER: Dave Berger from PPL Generation. My question is for Tim. You spoke about the operating costs of the solar being comparable to the operating costs of the conventional thermal power, and that struck me as a bit of a surprise. Normally, I think of fuel costs and the cost of air quality on the back end of a boiler as being part of the operating cost, and clearly you don't have that in a solar boiler. I was wondering what drivers there are. And one of the things you mentioned was cleaning mirrors, which never really occurred to me. But what are some of the drivers of operating cost?
MR. ZOLTOWSKI: If you think about the size of the heliostat field, cleaning is an ongoing process, so each mirror is cleaned once a day, but it's a continual process that happens pretty much 24 hours a day. The maintenance of the servo motors is probably one of the biggest costs. So when you look at -- I think there are 12,000 heliostats per field in that arrangement, so there are 24,000 per boiler. That particular facility has two power towers, so there are 48,000 servo motors that need to be maintained. And then you have the maintenance and operating costs that would be typical for the boiler.
MR. WODKA: Brian Wodka with RMF Engineering. You showed that using molten salt can expand in both directions steam generation. I understand that during the day with high solar energy you can store it and then it can extend outward. But in the morning if you don't have enough energy to boil water, how do you expand it over?
MR. ZOLTOWSKI: Good point. I think the theory is that there would be enough energy stored to do that in the morning. But you may be right. In practice it may be that it only extends it in the evening hours.
MR. BERGER: I think your point is that things don't cool as much, so you are starting at a higher level.
MR. ZOLTOWSKI: Right, you are starting at a higher level.
MR. WODKA: So it would last overnight?
MR. ZOLTOWSKI: Yes, that's the theory.
MR. BERGER: The salt is stored and it stays hot for a while. It doesn't cool as much as the working fuel (the water) would.
MR. ZOLTOWSKI: And I think there are some design techniques that may be applied in the near future. If you think about the construction of a power tower, it's designed so that it receives as much heat as possible. And in the nighttime, it actually reverses and you combine it with the air and it gives up as much heat as possible. So I think there might be some designs. These are just my thoughts. I haven't seen it out there, but there might be some way to protect it from heat loss when the sun goes down. I don't know if it would be some sort of retractable thing. I don't know. But it is interesting, when you think about it from a heat protection standpoint. I think Don mentioned how we can overheat the structures and then that puts structural integrity in jeopardy. Wind is a big factor. Just eighteen inches of movement will bring the structural component into focus of that energy. We heard earlier from our speaker about some of our space travel. It's the same technology that protects those structural components, the ceramic tiles as heat shields. There could be something similar to maintain heat over the nighttime. I don't know. I'm sure they will do research as this progresses.
DR. LAU: Normally your boiler would not be subject to cyclic loading every day. In this case, what happens if you have clouds coming in, going out, coming in again? Would that create a serious situation?
MR. ZOLTOWSKI: Yes, it would be a serious situation, and I think in most cases they would just shut it down for the day. That's why it's critical to pick a climate in which that type of transient would be minimized.
DR. LAU: So you would also monitor the loading that you would be facing?
MR. ZOLTOWSKI: That would be a management decision. It’s one of the things that prohibit this from being widely used from a commercial standpoint. It has to be a peak demand type situation or to lower energy costs at certain times, because it wouldn't be a continual generation and there are way too many things that interrupt it.